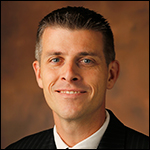
Joshua Caldwell, Flowers Family Chancellor’s Faculty Fellow in Engineering and associate professor of mechanical engineering, and Joseph Matson, a graduate student in Caldwell’s lab, have contributed to an international study that has discovered a new type of light-matter coupling. The work has long-term implications for how optical components can be even further miniaturized, a discovery that has potential to make a transformative shift in nanophotonic technology.
Optical components are created by blending materials that have varied functionalities, ranging from anti-reflection coatings and lenses to optical fibers. Traditionally these have been realized with refractive materials, with the refractive index derived from materials’ symmetrical crystal structure. But approximately a third of all minerals are known to have an asymmetrical, monoclinic lattice structure. This asymmetry modifies light propagation through a process called birefringence, while also offering opportunities to discover unexpected properties in the context of nanophotonics.
The researchers worked with beta-Gallium Oxide, a low-symmetry monoclinic crystal that has garnered interest as a wide bandgap semiconductor for power electronics and thin-film coatings of solar cells. Because of the monoclinic structure, when light couples with the polar bGO crystal the result is a “shear” force upon the resulting polariton—a half-light, half-matter quasi-particle.
Specifically, the international team discovered that a shear polariton’s propagation wavelength and direction depend on the infrared frequency and that their wave fronts are distorted with respect to higher symmetry crystals. “The discovery of these shear polaritons makes it possible to guide light in a material with unprecedented control of directionality and unique asymmetric propagation,” Matson said. “This level of control shows great promise for applications in on-chip photonics and compact infrared optical components.”
The researchers collaborated with researchers at the Fritz Haber Institute in Berlin, Germany, including Alexander Paarmann. The experiments were performed using the free-electron laser at the institute and featured collaborations with researchers including Thomas Folland from University of Iowa, Andrea Alù from the City University of New York and Mathias Schubert at University of Nebraska–Lincoln, along with students and postdocs from their teams.
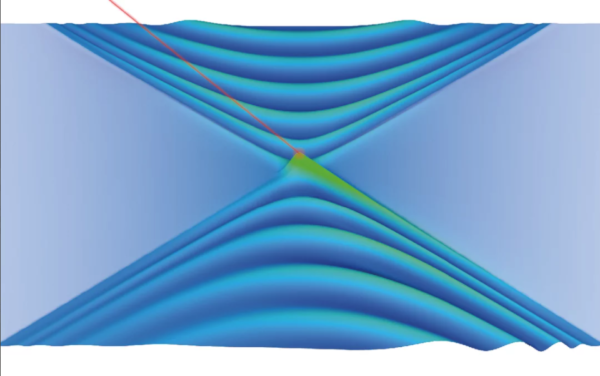
“The structure of the ‘monoclinic’ crystals used in our studies looks like a distorted cuboid, where four of six sides are rectangular but two are tilted parallelograms,” Paarmann said in a release. “Because of this distortion, the new shear waves not only run along well-defined directions across the crystal surface but are also no longer mirror-symmetric.”
“This work opens the door to exploiting this property within a broad range of low symmetry materials, including those with the triclinic system,” said Caldwell, also director of the Interdisciplinary Materials Science Graduate Program. “We will continue to study bGO, which offers a rich testbed of polaritonic behavior, as well as explore other monoclinic and triclinic crystals that are dominant in natural minerals to further develop the nanophotonic toolbox.”
The article “Hyperbolic shear polaritons in low-symmetry crystals” was published in the journal Nature on Feb. 23. Vanderbilt’s contributions to this research were supported by the Office of Naval Research grant no. N00014-18-12107 and the National Science Foundation Division of Materials Research grant no. 1904793.