A scientific team from Vanderbilt University and the Department of Energy’s Oak Ridge National Laboratory has made the first experimental observation of a material phase that had been predicted but never seen.
The discovery breaks new ground in the study of ferroelectricity, a characteristic of certain dielectric materials used in high-technology applications.
The team made the discovery using a layered, copper-containing crystal that is ferroelectric, or has a constant electric dipole that can be reversed when an electric field is applied. “These materials may become building blocks of ultrathin energy and electronics technologies,” said ORNL’s Nina Balke, a corresponding author of a paper reporting the finding in Nature Materials.
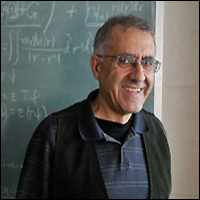
The theoretical research was carried out by the group of Sokrates Pantelides, University Distinguished Professor of Physics and Engineering at Vanderbilt and a Distinguished Visiting Scientist at ORNL for the last 25 years. Using quantum calculations, group members moved the atom responsible for polar displacement—copper—through the crystal structure and calculated the potential energy.
In experiments by Balke and Peter Maksymovych at ORNL, the team reports an undiscovered property known as a quadruple potential well, which plays a role in the mechanism known as ferroelectric switching–a process specific to ferroelectric materials which describes spontaneous reversible polarization through the application of an electric field, similar to the way atoms in magnets flip poles through the application of an external magnetic field.
“A typical outcome for a ferroelectric material is that you have two energy minima, or ‘wells,’ for this atom; each one represents a polarization vector, one pointing up, the other down,” said Pantelides. “For this material, theory predicted four energy minima, which is extremely unusual.”
Until now, physicists recognize only two wells as destinations for traveling atoms, which result in a binary record. The quadruple potential well increases the number of options in ferroelectric switching, yielding additional possibilities that could lead to increasingly complex operations and applications in data storage and electronics.
Pantelides’ group and several experimentalists at ORNL and other institutions performed a combination of calculations, simulations and experiments to verify the existence of the quadruple well, made possible by the complex layered structure of the material: ferroelectric copper indium thiophosphate (CuInP2S6), or CIPS. Sabine Neumayer at ORNL and John Brehm, Lei Tao and Andrew O’Hara at Vanderbilt were key participating postdoctoral scholars.
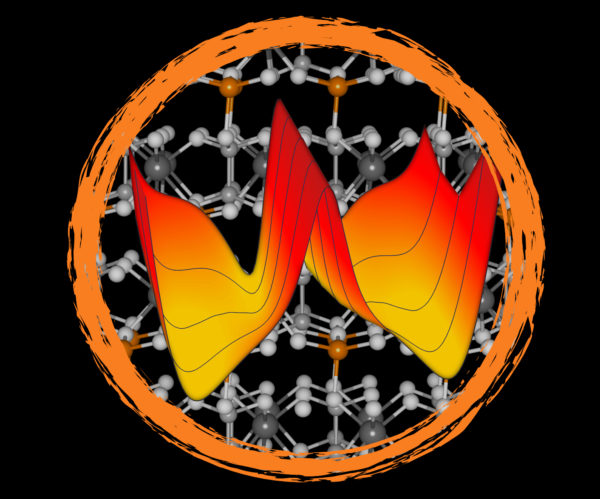
“This achievement is a powerful example of how integration of theory and experiment can lead to a major discovery,” said Pantelides, William A. and Nancy F. McMinn Professor of Physics and a professor of electrical engineering. “We worked together with our experimentalist collaborators at ORNL, who are experts in quantifying local piezoelectric material properties using scanning probe microscopy, and designed quantum calculations to probe how ferroelectricity works in CIPS.
“The calculations led us to a prediction of an unexpected feature with important consequences, and our friends designed just the right experiments to verify the predictions. Theory and experiment worked hand in hand from there,” Pantelides said.
The researchers ran experiments at ORNL’s Center for Nanophase Materials Sciences, where unsurpassed instrumentation and expertise enabled precise measurements and clear analysis and interpretation of complex data.
The experiments relied on piezoresponse force microscopy (PFM) to image and control ferroelectric domains on scales of millionths to billionths of meters. A sharp conductive probe applies an electric field to a sample’s surface, and the material’s electromechanically induced deformation is inferred from the probe’s displacement.
“CNMS is the world-leading institution in piezoresponse force microscopy,” said Maksymovych. “People come here from across the world to measure properties of their samples.”
A big draw is close consultation with PFM group members providing nearly half a century of cumulative expertise from innovators in PFM such as Sergei Kalinin and Stephen Jesse, and top names in theory, such as Panchapakesan Ganesh and Sokrates Pantelides, all authors on the paper: “Tunable quadruple-well ferroelectric van-der-Waals crystals.”
“Without that longstanding expertise, the measurement itself alone might have not resulted in the cohesive picture we gained,” Balke said.
In future studies, the researchers will probe dynamic properties—observing ratios of high and low polarization in strained materials; moving, stabilizing and embedding atoms of the new phase to make a switch; experimentally probing predicted behavior of materials under pressure; and studying how ferroelectric domains reorient after an electric field is applied.
Theory for the work is funded by the U.S. Department of Energy (grant DE-FG02-09ER46554) and by the McMinn Endowment at Vanderbilt University. The experiments were conducted at the Center for Nanophase Materials Sciences, which is a DOE Office of Science User Facility. Partial support for sample synthesis, experiments and theory was provided by the Laboratory Directed Research and Development program at the Oak Ridge National Laboratory.
Calculations were performed at the National Energy Research Scientific Computing Center, a DOE Office of Science User Facility supported by the Office of Science of the U.S. Department of Energy (contract No. DE-AC02-05CH11231). Manuscript preparation was partially funded by the Air Force Research Laboratory under an Air Force Office of Scientific Research grant (LRIR grant no. 14RQ08COR) and a grant from the National Research Council.
Contact: Brenda Ellis, (615) 343-6314, brenda.ellis@vanderbilt.edu
Compiled from Vanderbilt University and ORNL press releases